As someone new to CNCs, building a machine to meet my workshop needs came with a steep learning curve. I had to immerse myself in everything from CNC operation and troubleshooting to understanding the intricacies of designing and constructing one. Gathering this knowledge was a challenge in itself—I spent countless hours digging through hundreds, if not thousands, of sources to piece together a cohesive understanding of the subject matter.
What started as a simple attempt to document my CNC project for others who may want to build their own machine has evolved into something much larger than I originally anticipated. With that said, I apologize if the early articles seem overly detailed in their covering of background knowledge. I felt it was necessary to provide a thorough foundation, not just to prepare you for the more technical articles ahead but to equip you with a solid understanding so you won’t have to navigate the same scattered information I did. My hope is that this series of articles will save you time and effort, while helping you build both a CNC and your own knowledge with confidence.
Introduction
In our previous discussions, we highlighted the critical role of selecting the right spindle for your CNC machine. The spindle, often referred to as the heart of the machine, is essential for driving the cutting tool at the correct speed and torque to ensure precision and efficiency. We also explored key CNC build components, particularly those related to spindle performance, including spindle collets, end mills, flute types, end mill materials, and the differences between upcut and downcut mills—all essential topics for spindle selection. Additionally, we delved into machining time variables such as material removal rate, spindle speed, cutting speed, feed rates, and chip load, ensuring a comprehensive foundation before moving forward with more complex calculations and decisions.
Selecting the right spindle size and power is crucial to the success of any CNC project. This article is the first in a two-part series that will guide us through the process of determining the correct spindle sizing by analyzing power usage and spindle load across various milling operations. We will assess different materials, adjust cutting parameters, and examine power requirements to ensure an optimal cutting process.
In this first article, we will focus on defining the parameters for spindle selection. This involves identifying the relevant materials we’ll be working with, making informed assumptions, selecting an appropriate collet size, and choosing the most suitable milling operation. We will also describe the cutting scenario in detail, including the Axial Depth of Cut (ADOC) and Radial Depth of Cut (RDOC), and make careful choices for end mills. By the end of this article, we will have a clear framework in place that will allow us to perform calculations in the next article, where we will focus on analyzing spindle power and making final sizing recommendations.
Process Overview
The process of determining spindle power requirements involves a few structured steps to ensure accurate calculations and appropriate tool selection. Here’s the orderly approach we will be taking:
Establish Materials, Collet & Cutting Scenario
Before we can select the right end mill, it’s important to understand the material we are cutting (pine, walnut, aluminum, or steel). Knowing the material allows us to estimate cutting forces. We also need to decide on the collet size, ensuring it can handle the largest shank for stability, and select the type of cut we’re performing, which influences end mill selection and cutting forces.
Select the Appropriate End Mill
Once the material, collet size, and cutting scenario are defined, the next step is choosing the right end mill. Based on the material and desired operation, we select an end mill with the appropriate cutting diameter, shank size, flute count, and geometry (up-cut, down-cut, etc.). Choosing the right end mill ensures we can perform accurate calculations for power requirements that reflect the conditions of real life scenario.
Review Documentation and Fill Gaps
Once the end mills are identified, the manufacturer’s documentation usually provides critical data like maximum spindle speed, recommended feed rates, and chip load per tooth. The key here is that, if provided, we use the manufacturer’s values directly, eliminating the need for calculation. For missing values, if any, suppose cutting speed or feed rate, we rely on standard formulas to derive those numbers, using our knowledge from previous articles to ensure the accuracy of any calculated data.
In this approach, key parameters like spindle speed, cutting speed, and feed rate are only calculated when necessary, avoiding redundant work when the manufacturer provides reliable data. The goal is to efficiently determine spindle power based on a range of material removal rates and tool configurations.
Calculate Critical Parameters
With the documentation reviewed and any missing values filled in, we proceed to calculate three main cutting parameters that are essential for understanding the spindle power requirements:
Feed Rate (Inches per Second): While feed rate is traditionally provided in inches per minute (IPM), converting it to inches per second (IPS) can help better visualize how quickly the tool is moving across the material. This gives us a more intuitive sense of the cutting speed during real-time operations. If the feed rate is provided by the manufacturer, we simply convert it. If it’s not provided, we calculate it using chip load, spindle speed, and the number of flutes.
Material Removal Rate (MRR): The MRR is a crucial metric that tells us how much material is being removed during the operation, typically measured in cubic inches per minute. MRR gives us a quantitative understanding of how aggressively material is being removed, which is directly tied to the amount of power the spindle will need to handle the load.
Power Consumption (kW): Once we have the MRR, we calculate the spindle’s power consumption in kilowatts (kW). This is done by multiplying the MRR by the unit power for the material being cut (derived from previous tables or estimates) and dividing by a constant (1.341) to convert horsepower to kilowatts. This final calculation gives us a clear understanding of how much power the spindle will need to cut each material efficiently.
Test ADOC and RDOC Variations
After calculating base values, we explore different combinations of ADOC and RDOC. Testing a range of depths and widths of cut allows us to simulate different milling conditions and see how power consumption fluctuates. This step is critical for understanding the full range of spindle power requirements under different cutting loads.
Create a Power Consumption Chart
Finally, we consolidate the information and create a power consumption chart. This chart will visualize how changes in ADOC and RDOC affect spindle power across different materials. By plotting this data, we gain a clear understanding of the spindle power required for various scenarios, helping us select a spindle that is correctly sized for the demands of our CNC project.
The Spindle Sizing Paradox
The Paradox
One of the ironic challenges in determining the ideal spindle size for our CNC use case is the circular dependency between spindle size and end mill selection. Ideally, you need to know the end mill specifications, such as diameter and flute count, before finalizing your spindle selection. However, to choose the correct end mill, you first need an idea of the spindle size you plan to use. This paradox arises because the end mill’s compatibility is governed by the collet size, which itself is dependent on the spindle.
Unwinding the Paradox
Through previous discussions on collet sizes, we established that larger collet sizes are generally preferred over smaller ones due to their ability to hold larger end mill shanks. Larger shank sizes offer greater strength, stability, and durability during heavy cutting operations. Therefore, understanding the maximum collet size your spindle can support is crucial for optimal performance.
Also through our previous discussions on collets and spindles, we’ve established that spindles above 1.5kW are most appropriate for our needs. However, the drawback of a 1.5kW spindle is that it often comes with an ER11 collet, limiting us to a maximum end mill shank size of 1/4″. A 2.2kW spindle, on the other hand, offers more versatility by supporting an ER20 collet that can accommodate shank sizes up to 1/2″, doubling the strength and capability. If we consider a 3.5kW spindle, it would provide access to even larger collet options, such as ER25 or ER32, which support maximum shank sizes of 5/8″ and 3/4″ respectively.
While these larger collet sizes are tempting for their strength and versatility, the decision to go with a bigger spindle isn’t straightforward. A larger spindle may not be the best fit for my specific CNC requirements, especially when factoring in issues like increased machine rigidity requirements and potential vibration.
Approximating Collet Size
For this reason, I’ve decided to assume the largest reasonable collet size for this exercise – that is the ER20 collet, supporting a 1/2″ maximum shank size, for the remainder of this analysis. With this assumption, I will select appropriate end mills from available catalogs and use them to calculate the cutting forces and spindle requirements necessary for the operations we plan to perform. This approach helps us navigate the spindle sizing paradox and establish a more informed starting point for our CNC build.
Defining Relevant Materials
Soft Pine
Pine, a softer wood, is selected to highlight the differences in power demands compared to denser materials. Commonly used in light-duty woodworking projects, pine allows us to assess how the spindle manages simpler cuts. By comparing it with walnut, we can observe the power differences between working with softwood versus hardwood.
Black Walnut
Walnut, a much harder wood than pine, is frequently used in my custom furniture-making and chopping board projects. Including walnut in this study is crucial, as it directly represents the materials I work with regularly and will test the spindle’s ability to handle more demanding tasks.
Aluminum 6061
Aluminum represents our foray into metalworking. While softer than steel, it requires a distinct approach compared to wood. I’ve included aluminum because future projects will involve CNC machining custom aluminum parts. This makes it vital to understand the spindle power needed to machine aluminum efficiently and accurately.
Stainless Steel 316
Stainless steel serves as the extreme end of our comparison. Although I don’t expect to work with steel at all, its inclusion is valuable for understanding how spindle sizing changes when dealing with the hardest materials. Steel’s density and machining difficulty will illustrate how different material properties affect spindle power, especially when compared to softer materials like wood and aluminum.
Choosing The Definitive Milling Operation
While we have already explored the various milling operations and their power requirements in a previous article, it’s crucial to emphasize how these differences directly influence spindle sizing. Each milling operation places unique demands on the spindle, and understanding these demands is key to selecting the right spindle size for our CNC project.
For instance, engraving requires minimal spindle power due to its shallow cuts and low material removal rates, allowing smaller spindles to manage the task effectively. Conversely, operations like pocketing or slotting involve deeper cuts and engage more of the material, resulting in higher spindle loads. This variation in power requirements means that your spindle selection must align with the types of milling operations you plan to undertake, ensuring that it can handle both light and demanding tasks efficiently.
The Challenge
Selecting the most suitable milling operation for spindle sizing calculations can be challenging. The most power-intensive operations, such as slotting and plunging, engage the entire cutting tool and often do not provide flexibility in adjusting the Axial Depth of Cut (ADOC) and Radial Depth of Cut (RDOC). This limitation makes it difficult to fully understand how these variables impact the power requirements of the spindle since these operations don’t allow for gradual control or variation in cutting parameters.
On the other hand, moderate power operations like facing or pocket milling, or high power operations like side milling offer more control over ADOC and RDOC. However, they do not reflect the maximum possible power requirements that the spindle might face during rare, yet demanding operations. This creates a dilemma: Should we focus on operations that allow us to adjust key variables and gain more detailed insights, or should we choose more demanding operations that might set the upper limit for spindle power but lack the flexibility to explore those parameters?
To address this challenge, we can simulate high-power operations like slotting by mimicking 100% engagement of both ADOC and RDOC in controlled conditions. By doing so, we approximate the intense cutting forces a spindle would encounter in an actual slotting operation. This method provides us with a reasonably accurate picture of how the spindle might behave under high-load conditions, helping us make an informed decision about spindle sizing without being confined to the rigidity of actual slotting.
Side Milling
In this case study, I opted for side milling as the reference operation for the spindle sizing calculations. Side milling strikes a balance between adjustability and demand; it allows us to control the ADOC and RDOC, giving us insights into how these variables affect power requirements. At the same time, side milling is a high-power operation, as it involves removing a significant amount of material. This makes it more reflective of real-world scenarios where the spindle is subjected to considerable loads.
By choosing side milling, we ensure our calculations are robust enough to cover demanding situations while still providing the flexibility to explore different cutting parameters. This approach will help us determine a spindle size that can handle a wide range of operations, ensuring both versatility and reliability in our CNC build.
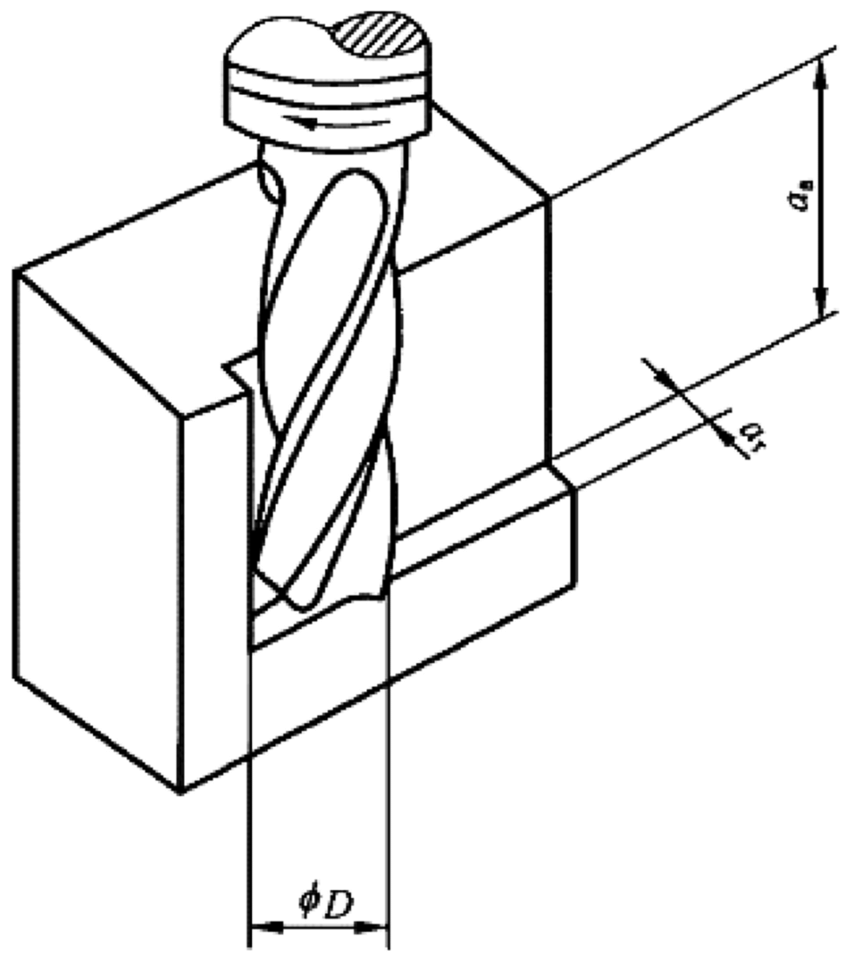
Describing The Cutting Scenario
For this spindle power calculation, we will focus on a roughing side milling operation on a 20″ x 3″ x 1″ workpiece. The objective is to pocket mill along the longest edge of the piece. While these dimensions are somewhat arbitrary for this exercise, they help you imagine the material to be milled and help provide a framework for adjusting the Axial Depth of Cut (ADOC) and Radial Depth of Cut (RDOC), which are essential for determining power usage during the operation. By experimenting with different materials—such as aluminum, walnut, and pine—we aim to explore how different material properties affect spindle power usage.
To ensure realistic conditions, we will begin by selecting appropriate end mills for each material and determining the recommended spindle speed for the chosen mills. If this information isn’t readily available, we’ll calculate spindle speed based on cutting speeds. Once we have the spindle speed, we can derive the ideal feed rate and, using the ADOC and RDOC, compute power usage and other critical factors.
The ADOC will be adjusted at 1/8″ each, and the RDOC will be adjusted at 1/16″ each until they reach their maximum cutting diameter and cutting height, allowing us to simulate different levels of tool engagement. This flexibility will help us see how changes in these variables influence the spindle’s power demand, particularly when switching between materials of varying hardness. For example, deeper cuts in aluminum will likely require significantly more power compared to softer materials like pine.
Choosing The Right End Mill
Selecting the right end mill is crucial to ensure optimal cutting performance and spindle power efficiency. After careful consideration, I sourced my end mill specifications from Amana Tool (Click Here to Visit) due to their comprehensive documentation and ease of product browsing. This choice simplified the selection process and provided a range of high-quality tools suitable for different materials and machining tasks.
Aluminum 6061
For aluminum milling, I opted for a high speed steel 3/8″ diameter double-flute spiral upcut end mill with a 1/2″ shank size, the largest shank size compatible with my assumed ER20 collet. The primary reason for choosing a larger diameter end mill is its ability to exert greater cutting forces, which is essential for this spindle sizing study. By selecting a tool that generates higher power demands, we can test the spindle under more challenging conditions, ensuring that our chosen spindle size can handle the worst-case scenarios during rough side milling.
An alternative would have been to use a 1/4″ diameter end mill, which could offer tighter cornering and higher precision. However, the purpose of this calculation is not to focus on fine details but to evaluate the spindle’s ability to handle demanding operations. A smaller end mill would have a thinner profile, increasing the risk of deflection and wear during rough milling, leading to reduced surface quality and potential tool breakage. Additionally, using a smaller tool would necessitate multiple passes to clear the same material volume, increasing the load variability on the spindle. The 3/8″ diameter end mill, on the other hand, provides a more rigid profile and allows for deeper and wider cuts. The 1/2″ shank offers additional stability, reducing the likelihood of tool deflection.
The choice of a double-flute design was also intentional. A double-flute end mill provides an excellent balance between chip clearance and cutting speed. In aluminum milling, effective chip evacuation is crucial to avoid clogging and overheating, which can compromise tool performance and surface quality. The upcut spiral direction was chosen to actively pull chips away from the cutting area, enhancing the surface finish and reducing tool wear during the side milling process. By selecting this 3/8″ diameter tool with a 1/2″ shank, we aim to test the spindle’s capability in handling high-force, high-demand scenarios effectively.
Pine & Black Walnut
For this side milling operation on wood, I selected the Amana 46420-K Solid Carbide Coated Spiral Plunge Down-Cut End Mill, which has a 3/8″ diameter and a 3/8″ shank. Unlike the end mill chosen for aluminum, where I prioritized a larger 1/2″ shank for added rigidity, I had to adjust my selection for wood. Most wood-specific end mills, including those from Amana, have matching cutting and shank diameters. Although my initial intention was to maintain consistent shank sizes across all material tests, the unavailability of 1/2″ shank end mills designed for wood, which also had a 3/8″ cutting diameter led me to choose this 3/8″ shank option with a 3/8″ cutting diameter. I felt it was more necessary to match the cutting diameter than the shank diameter for comparative reasons.
Despite the smaller shank, this end mill provides the necessary strength and accuracy for the rough pocket milling tasks on wood. This choice ensures that my tests across different materials, including pine and walnut, remain consistent as much as possible, while also reflecting the specific needs of wood milling. Ultimately, this decision allows for accurate spindle power calculations across a range of materials.
I also initially leaned toward selecting a single-flute end mill due to its superior chip evacuation. Single-flute end mills are particularly effective for milling wood because they provide more space for clearing chips, reduce heat buildup, and allow smoother cuts. However, I couldn’t find a single-flute end mill that matched my specific criteria, particularly the 3/8″ cutting diameter and a design tailored specifically for milling wood. As a result, I opted for a double-flute end mill, which, while not ideal for chip clearance, offered the right balance of performance for wood without compromising other important factors like diameter and shank size.
A key factor in my decision was the down-cut spiral design of the selected end mill. While an up-cut mill excels at pulling chips away from the cutting area, it can cause significant tear-out in wood, especially in softer woods like pine. To avoid tear-out and ensure a cleaner surface finish, I chose a down-cut end mill, which presses wood fibers downward as it cuts, preventing splintering and preserving the material’s integrity. This design is also beneficial when working with harder woods like black walnut, as it helps maintain clean edges and reduces the risk of cracking or damage during the milling process.
Stainless Steel 316
For the side milling operation on steel, I selected the Amana 51607 Upcut Helix Spiral Corner Radius Bottom of 3/8″ diameter, 3/8″ shank end mill. My goal was to maintain consistency in the cutting and shank diameters, which is why I kept both at 3/8″—the same as the end mills chosen for wood and aluminum. This ensures that the comparison of spindle power requirements across the three materials—steel, wood, and aluminum—remains consistent, allowing us to observe the differences in performance and power demand more clearly.
One noticeable difference in this selection is that I wasn’t able to find an end mill with the same cutting height as the 1-1/4″ end mills used for wood and aluminum. While this was something I had initially hoped to match, it wasn’t the most critical factor for this test, as I prioritized the end mill’s ability to withstand the hardness of steel over the length of the cutting flute. The shorter flute length will still allow me to engage the material effectively while minimizing deflection in such a hard material.
Additionally, this end mill features a 4-flute design, which stands in contrast to the two-flute options chosen for wood and aluminum. The higher flute count is necessary for steel due to its increased strength, as more flutes contribute to the overall rigidity of the tool. This design increases the tool’s strength and reduces chatter, ensuring that the end mill can tackle the harder properties of steel without compromising on performance.
Overall, this end mill balances strength, durability, and cutting efficiency for steel, though I don’t plan to mill steel with my CNC. Its inclusion is purely for study purposes, providing a valuable comparative baseline against wood and aluminum. This will help assess the spindle’s power requirements when switching between materials with significantly different hardness and properties.
Next Steps
Now that we’ve established the materials, collet size, and cutting scenario, and selected the right end mill for each material, the next phase involves diving deeper into the calculations. In the following article, we will proceed with determining critical cutting parameters such as feed rate, material removal rate (MRR), and spindle power consumption. Additionally, we will explore how variations in Axial Depth of Cut (ADOC) and Radial Depth of Cut (RDOC) affect the power requirements. Finally, we’ll use this data to create a power consumption chart, giving us a comprehensive understanding of the spindle power needed under different cutting conditions to ensure the CNC setup is properly configured.